Filter presses have a wide range of types and models. The typical filter continue the market are plate and framework filter press, chamber filter presses and membrane layer filter presses according to the existing sludge treatment requirements. So what is the distinction between these 3 filter presses?
Briefly
Merely talking, plate and structure filter presses, chamber filter presses, and membrane layer filter presses are all plate and structure filter presses.
1. Plate and framework filter press– one plate and one structure as a group, is the oldest kind
2. Chamber filter press– mud cake developed in the filter box
3. Membrane layer filter press– a box plate + a diaphragm plate
When it comes to the equipment type, there are three types: upright, high cantilever, and side bars. This relates to just how the filter board is connected to the device.
Whether the fully automatic plate and frame filter press, completely automated chamber filter press or high pressure diaphragm filter press, the working concept of theirs all pressure filtration, which is the same working concept of filter press. The necessary difference in between plate and framework filter press, chamber filter press and diaphragm filter press is that the differences in filter plates utilized. Through the filter plate to distinguish which kind of filter press, according to the use of demands to select the filter press.
In the professional
Various filter plate structure
The filter chamber of plate and frame filter press is formed by intertwining 2 kinds of filter plates, a strong filter plate and a framework filter plate. Each filter press has a various number of plate frameworks depending on the dimension of area. Plate and framework filter presses are normally utilized for product purification with much less granular objects and fine items.
Chamber filter press is composed of 2 the same filter plates to develop a filter unit. When the two filter plates are pressed together, a filter chamber is formed for saving the granular things separated by the filter cloth. The feed hole is placed in the facility of filter plate. The single filter plate is thicker than home plate and framework filter plate. Usually used for products with even more granular objects, such as sewer treatment, mineral processing, coal washing, etc.
The filter plate made use of in membrane filter press has a diaphragm tooth cavity on both sides. There will be inlet holes on the side of filter plate. It’s hollow in the center, and it’ll appear when it’s blown up. The plate and structure filter press and diaphragm filter press do not have this function, which is really various.
Different press cloth designs
Home plate and framework filter press filter fabric is a square filter cloth, which can be straight put in between the filter plates.
The diaphragm filter press applies two filter cloths connected in the center to create a filter cloth system. Among the two layers is travelled through the middle opening of the filter plate and then squashed to cover the filter plate.
Various filter tooth cavity formation
The filter chamber of plate and framework filter press requires 2 solid filter plates plus a structure filter plate. The diaphragm filter press consists of a strong filter plate and a hollow filter plate.
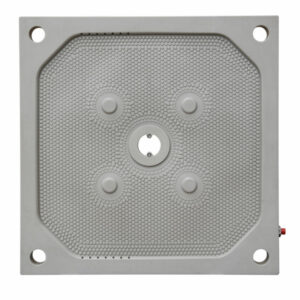